Into the Quantum Realm
Department of Energy selects W&M physicists to advance quantum computing
May 23, 2022
By
Adrienne Berard
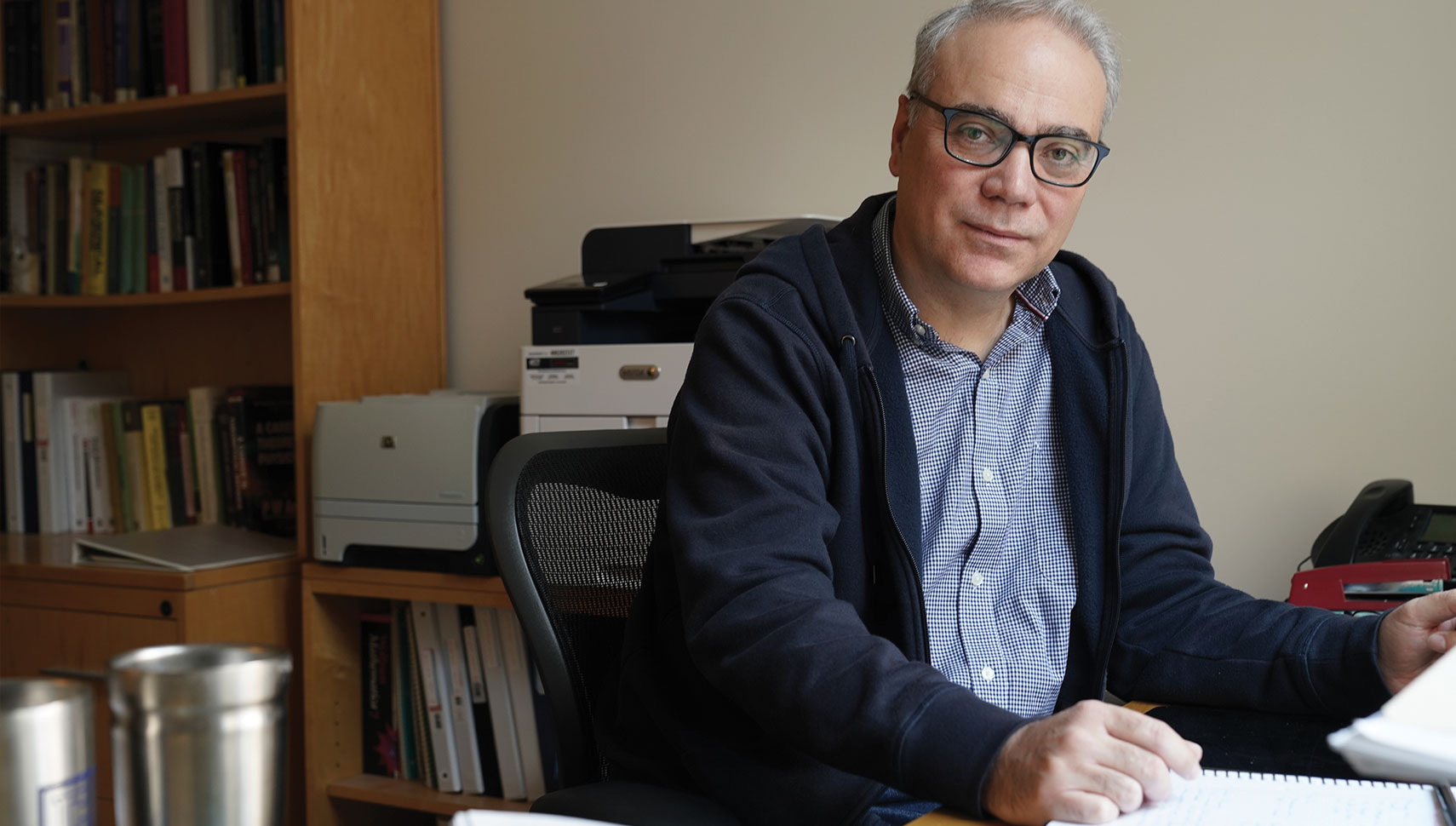
The computers and sensors of the future are being engineered one atom at a time. A team of theoretical quantum physicists at William & Mary has partnered with materials scientists to develop a new tool for harnessing the power of subatomic conductivity.
“Our role as theoretical physicists is to think about how to choose the different parameters of an experiment,” said Enrico Rossi, associate professor of physics at William & Mary, who leads a Condensed Matter Theory group at the university. “In this case, we wanted to know how to tune a nanoscale device to basically be in a condition where its state of superconductivity makes it a very sensitive sensor.”
The project, done in collaboration with material scientists Wei Pan of Sandia National Laboratories and Javad Shabani of New York University, aims to address a major hurdle on the path to a more advanced quantum computer — development of sensor-like structures capable of encoding a quantum bit, the equivalent of the ones and zeros used in today’s binary computing language.
Rossi’s collaboration was one of 29 projects selected by the U.S. Department of Energy (DOE) to receive a portion of $73 million in funding to advance quantum information science (QIS) research to develop the next generation of quantum smart devices and quantum computing technology. “Quantum science represents the next technological revolution and frontier in the Information Age, and America stands at the forefront,” U.S. Secretary of Energy Jennifer Granholm said in a release. “At DOE, we’re investing in the fundamental research, led by universities and our National Labs, that will enhance our resiliency in the face of growing cyber threats and climate disasters, paving the path to a cleaner, more secure future.”
Solving the world’s biggest problems starts small — very small. Rossi explained that his team is developing theory for semiconductor-based technology that their collaborators at NYU and Sandia are able to build at the scale of a single atom.
“They use what’s called molecular beam epitaxy and what that means is that they grow this material one atom at a time in a perfectly clean environment,” Rossi said. “By doing that, they can control very carefully the thickness and make sure that there are no impurities flying in and interfering with the structure.”
The ultimate goal is to have the structure so sensitive to interference that a single photon will set it off, Rossi explained. The end product will be what’s called a topological superconducting device, a specific combination of superconductors and semiconductors layered together in such a way that the slightest interference — for instance, a single photon — will push the structure into a topological superconducting state.
“We need the material to be extremely close to a transition between being a normal superconductor and a topological superconductor,” Rossi said. “When they’re so close to this transition, they’re very sensitive to external perturbations, which means they function as sensors. On the other hand, a system firmly in the topological superconducting phase can be very impervious to external perturbations, a fact that makes it ideal to realize robust quantum bits.”
Rossi likens the material to a bag of supercooled water. When water is clean enough, it can be carefully cooled below the normal freezing point. It’s at freezing temperature but remains liquid.
“It’s very unstable, very ready to become all ice,” Rossi explained. “In fact, if you drop in one single grain of salt, the molecules immediately nucleate and there’s instant crystallization. It suddenly becomes ice.”
He explained that a system that is just at the edge of the transition between one state and another is an excellent way to monitor changes in an environment.
“In our situation, we think that we can tune the system to be very close to the transition between being a normal superconductor and a topological superconductor — so close in fact that an external photon can basically drive the system into another phase,” Rossi said.
Rossi explained that the team’s device will be able to sense slight changes in an electromagnetic field, allowing for the development of telescopes with the power to sense light that is no longer visible or stronger electroencephalograms (EEGs), able to detect abnormalities in brain waves by sensing single neurons.
“Let’s say you have a radio that’s really far away from the output source,” Rossi said. “If you can’t pick up the radio waves, then you can’t listen to it anymore. But if you have a very sensitive radio, you can keep moving hundreds of miles away from the source and still listen to those radio waves, even if they are very, very weak. That’s what we’re doing with this device.”
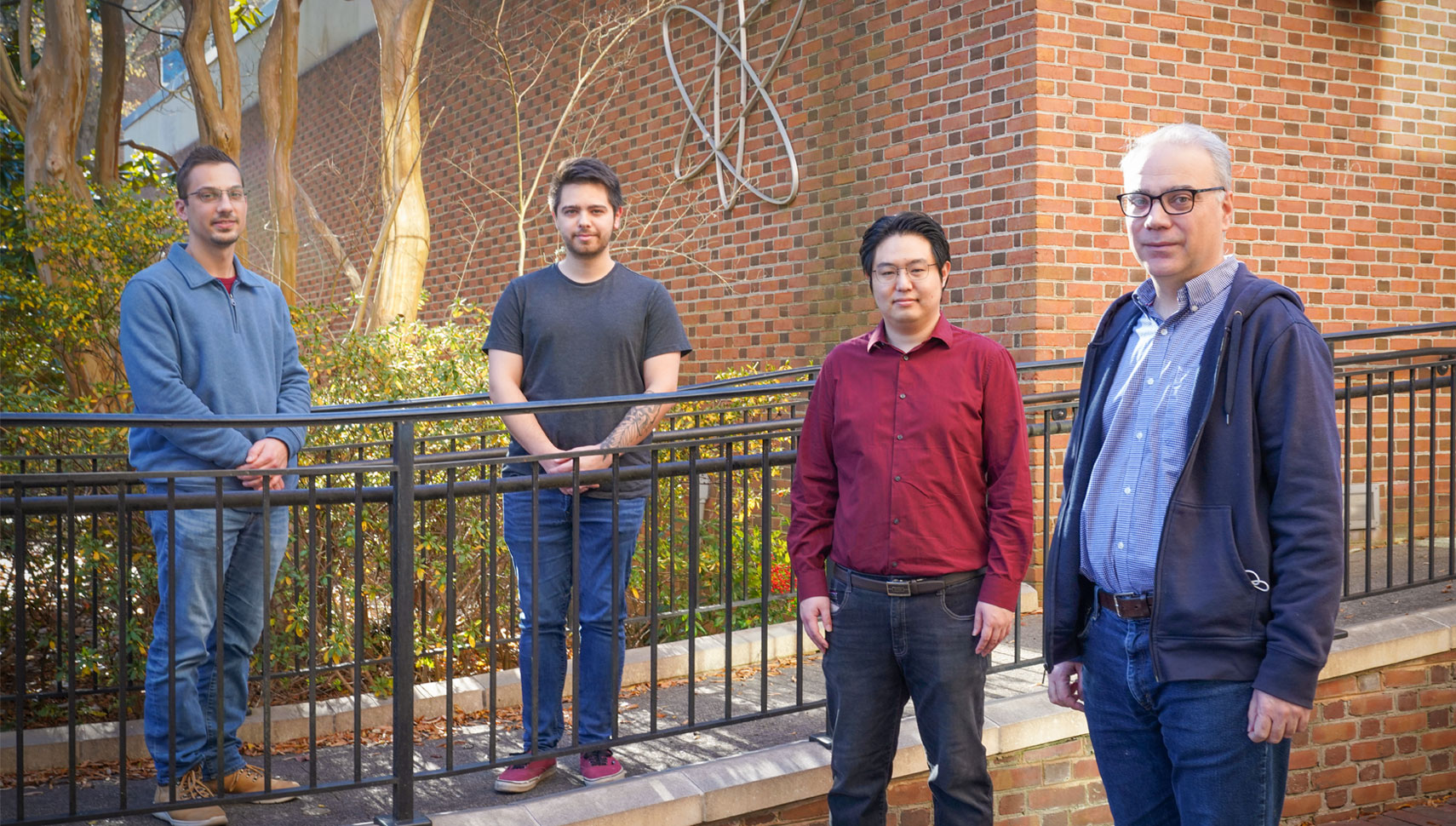