Applied Science
Ph.D. students explain their cutting-edge research and the science equipment that makes it possible
February 13, 2024
By
Carlos A. da Silva Jr.
and
Andriana C. "Andrea" Zourou
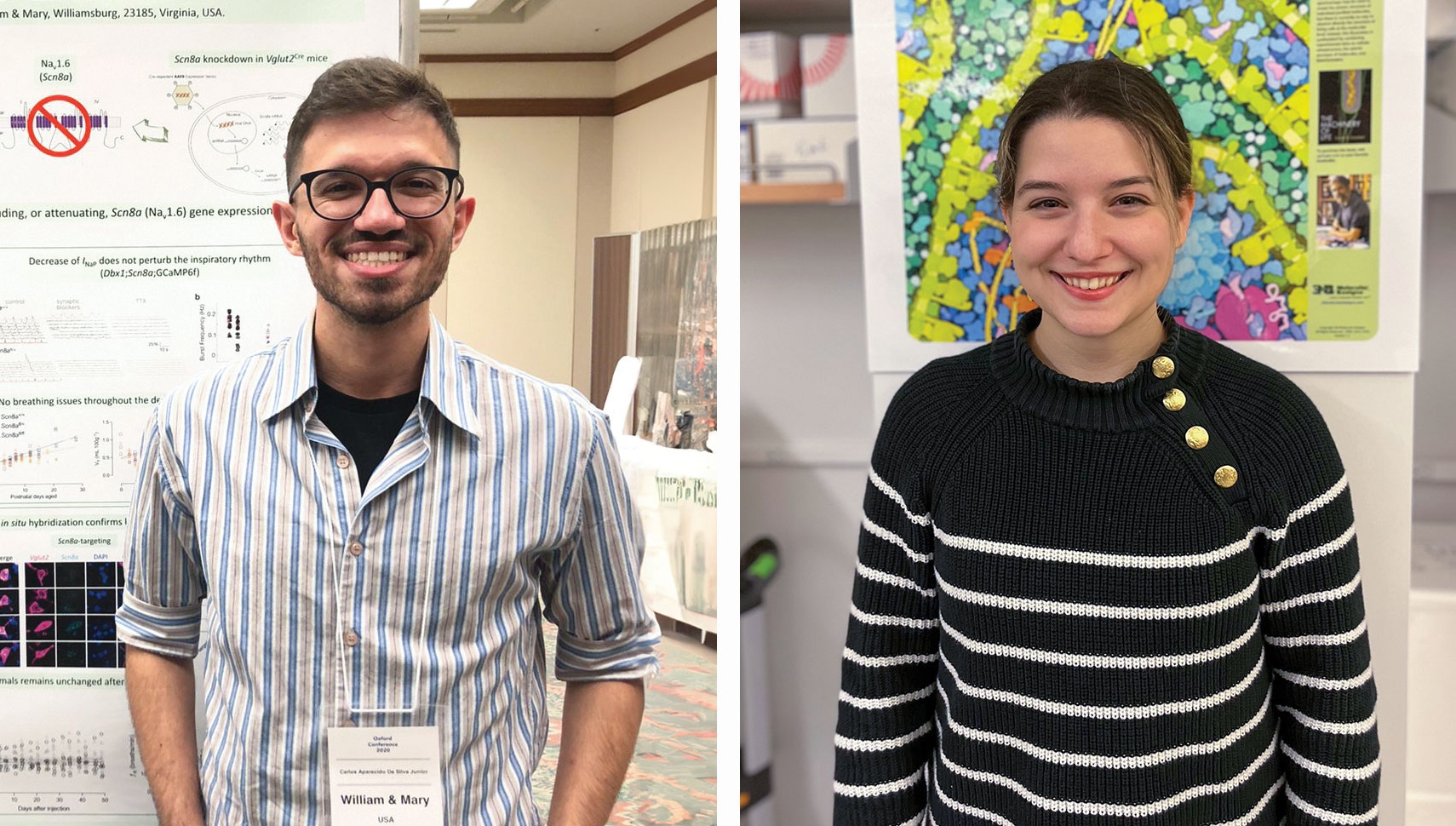
Applied science at William & Mary is an interdisciplinary graduate department that uses tools, techniques and principles from multiple sciences to solve complex scientific and technical problems. Graduate students work with faculty from departments throughout the university, as well as with partners from Jefferson Lab, NASA Langley and others.
In November, the Board of Visitors voted unanimously to approve a proposal for a new school at William & Mary that will include the applied science, physics, computer science and data science departments and programs. Master’s and doctoral degrees in applied science, computer science and physics made up over one third of all the graduate degrees conferred by Arts & Sciences in 2023, and of the top 30 most-cited W&M authors, more than half (54%) hail from computer science, data science, applied science and physics. Through the new school, William & Mary plans to expand its focus on data fluency — a pillar of the university’s Vision 2026 strategic plan — facilitating data-intensive research while further enhancing students’ career prospects in sectors that are driving economic growth. A final proposal will be submitted to the State Council of Higher Education for Virginia (SCHEV) by March 2024 and if approved, the school would launch in fall 2025.
We asked two current doctoral candidates in applied sciences to describe their research on breathing physiology and molecular interactions and explain the scientific equipment that facilitates it.
‘A BACKSTAGE PASS TO THE LUNG SYMPHONY’
By Carlos A. da Silva Jr.
Hey there, lung enthusiast!
While reading this article, you will probably take about 36 breaths. As for me, I took so many while writing this that I lost count. But fear not! You and I do not need to worry about counting each breath, or even think about keeping them going. Your brain has turned on the autopilot and is taking care of that for you. So, lean back, grab a coffee and enjoy this riveting respiratory roller coaster.
Now picture this: 12 breaths per minute are what an average, healthy, 150-pound human would take. Curiously, if a mouse could also read this article, it would be hyperventilating at a whopping 200 breaths per minute.
So, how do we unravel the mysteries of breathing physiology? Here in the Department of Applied Science at William & Mary, I developed a system that allows us to record respiratory parameters in small animals for long periods. It’s based on a methodology and equipment that was already being used, but I refined it to be more accurate and take additional measurements.
This technique is called “whole-body plethysmography,” and here’s some science. The animal is placed in a chamber connected to a pressure transducer, with air flowing through all the time. During each breath, the animal inhales and exhales, causing changes in the temperature and pressure inside the chamber, which I track. At the same time, I can sample the oxygen content in that chamber. It’s a mouse-sized VIP lounge.
After crunching some numbers, I obtain different parameters such as its respiratory frequency and its oxygen consumption (used to make inferences about the metabolic rate).
If you wondered why that is important, I’ve got the answer for you. The lab where I am working toward my Ph.D. is dedicated to understanding how the brain orchestrates our respiratory symphony, uncovering the molecular basis of this behavior, and how it can be influenced and changed when exogenous factors are present.
Imagine this: Everything is working rhythmically and involuntarily. However, things can go haywire. Therefore, understanding the neurophysiology of breathing in mammals has a translational value as it adds knowledge that helps neuroscientists elucidating respiratory dysfunctions in premature and full-term babies, children and adults, and those who have neurodegenerative disorders.
Unfortunately, I can’t help you with studying the brain of a mouse that reads, but I certainly have the tools if you are curious about studying the intricate paths your brain takes to keep you breathing. It’s like a backstage pass to the lung symphony — minus the mice in tuxedos.
Carlos A. da Silva Jr. is a Ph.D. student in the W&M Department of Applied Science, working in the neuroscience lab with Professor Christopher Del Negro.
PROBING MOLECULAR INTERACTIONS WITH
NUCLEAR MAGNETIC RESONANCE
By Andriana C. “Andrea” Zourou
Nearly 80 years ago, American physicist Isidor Isaac Rabi discovered that nuclei act as small magnets when placed in a magnetic field and won the Nobel Prize in physics in 1944. Since then, the field of nuclear magnetic resonance (NMR) has evolved with giant leaps and has applications in clinical diagnostics, drug development and materials science.
In my doctoral research, I use NMR to probe the relationship between small proteins and lipids — building blocks of living matter — as they interact in the solid state. The goal is to identify antimicrobial and anti-inflammatory agents with the potential for development into therapeutics.
A collaboration between William & Mary and the National High Magnetic Field Laboratory in Tallahassee, Florida, which is home to the world’s largest and most high-powered magnet lab, enables me to obtain data from a 14.1-tesla (600-megahertz) instrument on samples I prepare at W&M. For comparison, MRI machines typically have a magnetic field strength of only 0.2-3 tesla, which are units of magnetic flux density.
Nestled inside two “jackets” of cryogenic coolants, a layer of liquid helium and one of liquid nitrogen, a powerful superconducting magnet gives rise to a magnetic field that is most homogeneous at the very center of the instrument. There, the lipid and peptide samples are inserted with a probe. The superconducting coils that surround them exert energy of different radio frequencies (RF). Specific types of nuclei absorb at different RF energies that are unique to their identity. The strength of the magnetic field at the center of the magnet makes the nuclei rotate in a way that is characteristic of how they interact with their environment. Depending on how we apply the RF pulses, this precession gives rise to electromagnetic signals that are captured by the detector. From that, we can determine the molecular interactions at play in the samples.
With the 600-MHz magnet that is typically used for my samples, I measure the insertion of peptides with antibiotic properties inside lipid bilayers that mimic cell membranes. The goal is to elucidate mechanisms of antimicrobial action and identify synergistic agents, such as other peptides or specific lipids, that favor these interactions. Solving mechanisms of drug actions is part of a wider effort of rational drug design in the 21st century using a bottom-up approach that will result in more effective drugs with fewer side effects. NMR is one of the most powerful techniques at the forefront of this effort and by having access to it, researchers like me at William & Mary can push the boundaries of what it is possible to understand at the atomic level.
Andriana C. “Andrea” Zourou is a Ph.D. student in the W&M Department of Applied Science, working in the biophysical chemistry lab with Professor Myriam Cotten.